Research
The broad area of my research lies in the field of Cosmology which is the study of the evolution of the universe as a whole. I mainly focus on the formation of first structures and luminous objects like stars and galaxies in the high redshift universe. I also try to understand how these new sources of energy influence the thermal and ionization histories of the universe as a whole. I use large-scale cosmological simulations based on the standard ΛCDM cosmology to generate a small realization of structure formation in the universe and compare their behaviour with observations.
Cosmic Dawn
The lighting up of the first generation of galaxies has captivated the attention of scientists for a long time. The discovery of these ”islands of stars” by Edwin Hubble in the 1920s, along with the expansion of the universe, marked the beginning of Precision Cosmology. Observing the galaxies has always been a probe into the physics of a much deeper and earlier universe. The epoch of the formation of self-gravitating structures leading to the first stars and galaxies in the universe’s cold, dark, neutral, and expanding background is called the Cosmic Dawn.
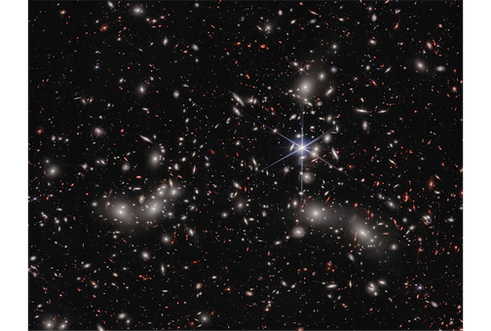
Epoch of Reionization
New stars and galaxies form in the dense cores of DM halos, but their impact is far-reaching, influencing the entire universe. The energetic photons released from these new sources and their dramatic remnants have the potential to ionize the whole universe once again. This second global phase transition of the universe (after recombination) is called the Epoch of Reionization. If we try to picture the universe, we would see a growing transparent bubble of ionized gas around each source in a background of opaque neutral gas, looking like Swiss Cheese. Over time, these ionized bubbles grow in size and overlap to cover the entire space.
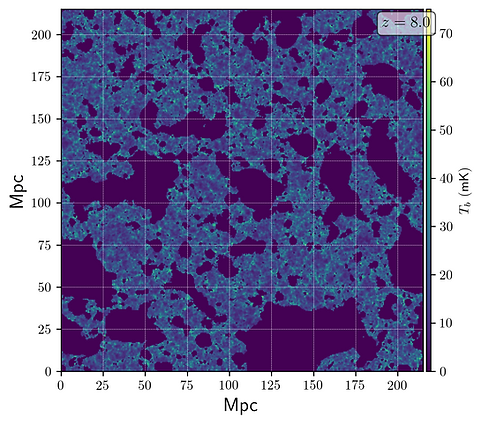
21 cm Cosmology
After the Recombination, the universe was mostly neutral hydrogen and CMB, but they did not interact with each other since the CMB did not have enough energy to excite the hydrogen to higher states. However, it was soon discovered that hydrogen could interact with photons of much lower energy by flipping the spin of the electron with respect to the spin of the proton in hydrogen. Using the mathematics of spin quantum mechanics of hydrogen and taking into account the finite radius of the proton, we find that the state where the spins of the proton and electron are aligned (triplet state) has higher energy compared to the state when the spins are opposite (singlet state). This is known as the Hyperfine splitting of the ground state of the hydrogen atom. Although this picture of aligned spins gives a very vague description of the actual quantum scenario, the mathematics shows that a photon of wavelength 21 cm is emitted to flip a spin from the triplet state to a singlet state. This process causes the neutral hydrogen gas to glow dimly. The 21 cm line directly follows the neutral hydrogen density in EoR and can explore the nature and evolution of the first generation of galaxies. It can also potentially monitor the large-scale effects of luminous sources (such as heating) by their absorption and emission signatures.
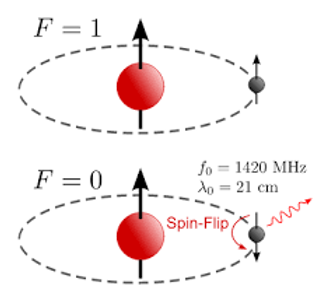
Cosmic Web
Figure 4 shows the distribution of galaxies in space. It clearly shows that some galaxies are located in high-density clusters containing several hundreds or smaller groups having a few to tens of galaxies. The majority of all galaxies, however, are distributed in low-density filamentary or sheet-like structures. These sheets and filaments surround large voids, regions with diameters up to ∼ 100M pc that contain very few galaxies. This Cosmic Web is a direct consequence of the asymmetric non-linear collapse of matter, which divides the universe’s matter density into different environments like voids, sheets, filaments and clusters/knots. It is also essential to properly quantify the galaxy environments because specific observed properties of galaxies may correlate with them. For example, the morphology density relation stipulates that elliptical galaxies are preferentially in crowded environments and spiral galaxies in the field. The same kind of correlation can be found in the colours of the galaxies, star formation history and ages.
